Our work has demonstrated that metal complexes can serve as catalysts to promote the conversion of CO2 into organic compounds via electrochemical reduction. The catalytic activity of these materials and their immobilization on solid surfaces can both be tuned based on molecular design. Catalytic activity under visible light has been achieved using a hybrid photocatalyst or a photoelectrode that combines the functions of a photoactive semiconductor and a metal complex catalyst. In this hybrid photosystem, the semiconductor absorbs visible light, following which the resulting photoexcited electrons are rapidly transferred to the catalyst at rates on the picosecond time scale. CO2 is then selectively transformed to generate organic compounds via reduction in solution.
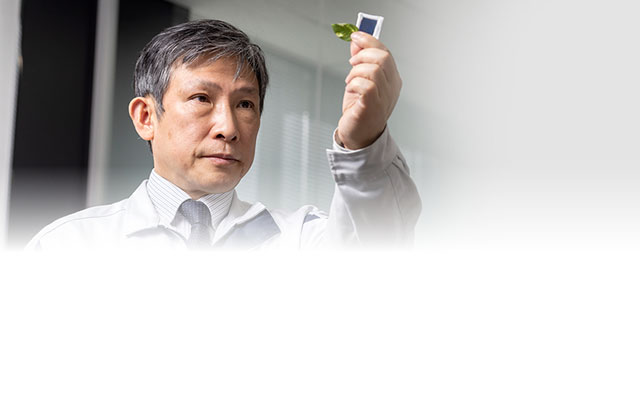
Research
The photosynthesis that occurs in plants generates organic compounds from water (H2O) and carbon dioxide (CO2) using sunlight, such that solar energy is stored in the form of chemical energy.
Our group has researched artificial photosynthesis systems capable of proceeding under visible light irradiation, in which electrons (e-) and hydrogen ions (H+) are extracted from water,
leaving dioxygen (O2). These species are subsequently involved in the reduction of CO2 to synthesize organic substances. We have previously demonstrated a new method that combines
the unique characteristics of a metal complex catalyst (intended to promote the reduction of CO2),
an inorganic catalyst (for the oxidation of water) and a semiconductor (for visible light absorption).
As an example, a so-called artificial leaf device was shown to store solar radiation in the form of chemical energy by producing formic acid (HCOOH),
with a conversion efficiency exceeding that of plants.
At present, we are conducting research with the aim of creating viable artificial photosynthetic systems capable of creating new energy carriers with minimal environmental impact.
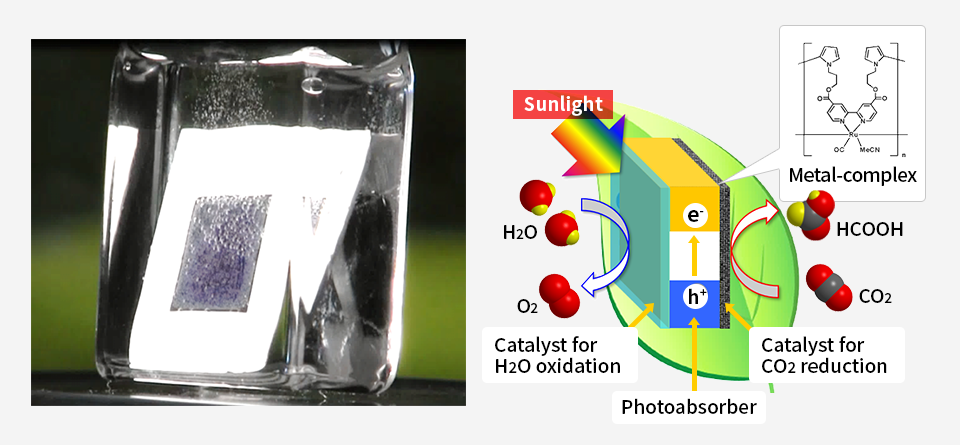
- 1. Photocatalysts and photoelectrodes combining semiconductors and metal complex catalysts
-
- (1)Morikawa, T., et al., Applied Physics Letters, Vol. 96 (2010), 142111-1~3.
https://doi.org/10.1063/1.3386532 - (2)Sato, S., Morikawa, T., et al., Angewandte Chemie-International Edition, Vol. 49 (2010), pp. 5101-5105.
https://doi.org/10.1002/anie.201000613 - (3)Arai, T., Sato, S., et al., Chemical Communications, Vol. 46 (2010), pp. 6944-6946.
https://doi.org/10.1039/c0cc02061c - (4)Suzuki, T. M., Nakamura T., et al., Journal of Materials Chemistry, Vol. 22 (2012), pp. 24584-24590.
https://doi.org/10.1039/c2jm33980c
- (1)Morikawa, T., et al., Applied Physics Letters, Vol. 96 (2010), 142111-1~3.
- 2. Artificial leaf devices
-
Our prior work developed a hybrid monolithic photoelectrode comprising a semiconductor and a metal complex catalyst and having a tablet construction, referred to as an “artificial leaf.” When immersed in an aqueous solution containing CO2, this device was able to generate HCOOH from CO2 and H2O in response to irradiation with sunlight. During this process, one side of the electrode generated formic acid while bubbles of oxygen were produced from the opposite side. This artificial leaf functioned with a solar-to-chemical energy conversion efficiency of 4.6%, which exceeds the photosynthesis efficiency of plants. This highly efficient artificial leaf cannot be constructed simply by conventional combinations of various materials. Our newly-developed catalyst technology, which proceeds CO2 reduction reaction at a low electrical potential close to the theoretical limit, contributes to the artificial leaf.
- (1)Sato, S., Arai, T., Morikawa, T., Uemura, K., Suzuki, T.M., Tanaka, H. and Kajino, T., Journal of the American Chemical Society, Vol.133 (2011), pp. 15240-15243.
https://doi.org/10.1021/ja204881d - (2)Arai, T., Sato, S., Kajino, T. and Morikawa, T., Energy & Environmental Science, Vol. 6 (2013), pp. 1274-1282.
https://doi.org/10.1039/c3ee24317f - (3)Arai, T., Sato, S. and Morikawa, T., Energy & Environmental Science, Vol. 8 (2015), pp. 1998-2002.
https://doi.org/10.1039/c5ee01314c
- (1)Sato, S., Arai, T., Morikawa, T., Uemura, K., Suzuki, T.M., Tanaka, H. and Kajino, T., Journal of the American Chemical Society, Vol.133 (2011), pp. 15240-15243.
- 3. Artificial photosynthesis with photocatalytic particles suspended in water
-
Techniques in which the CO2 reduction reaction is promoted by photocatalyst particles suspended in aqueous solution are expected to allow the realization of practical, low cost and scalable artificial photosynthesis systems. In these systems, a complex catalyst/semiconductor hybrid photocatalyst reduces CO2, a semiconductor photocatalyst oxidizes water, and another metal complex mediates electron transfer between these two types of particles. All these materials are suspended in an aqueous solution. In previous joint research, the first-ever visible-light-driven particle suspension system for CO2 reduction was realized. In this system, the competitive hydrogen generation reaction was suppressed even in water, with the simultaneous generation of dioxygen. This system also recently exhibited a reaction rate that was increased by an order of magnitude.
- (1)Suzuki, T. M., Yoshino, S., Takayama, T., Iwase, A., Kudo, A. and Morikawa, T., Chem. Commun., Vol. 54 (2018), pp. 10199-10202.
https://doi.org/10.1039/c8cc05505j - (2)Suzuki, T. M., Iwase, A., Tanaka, H., Sato, S., Kudo, A. and Morikawa, T., Journal of Materials Chemistry A, Vol. 3 (2015), pp. 13283-13290.
https://doi.org/10.1039/c5ta02045j - (3)Morikawa, T., et al., ChemSusChem, Vol. 12 (2019), pp. 1807-1824.
https://doi.org/10.1002/cssc.201900441 - (4)Suzuki, T. M., Takayama, T., Sato, S., Iwase, A., Kudo, A. and Morikawa, T., Applied Catalysis B: Environmental, Vol. 224 (2018), pp. 572-578.
https://doi.org/10.1016/j.apcatb.2017.10.053
- (1)Suzuki, T. M., Yoshino, S., Takayama, T., Iwase, A., Kudo, A. and Morikawa, T., Chem. Commun., Vol. 54 (2018), pp. 10199-10202.
- 4. CO2 reduction systems utilizing Earth-abundant elements
-
In order to fully determine the reductions in CO2 emissions associated with using artificial photosynthesis, a life cycle assessment of all aspects of the system is required, including manufacturing and operation. The use of Earth-abundant elements is also important. For this reason, we are presently researching semiconductors and catalysts that are primarily composed of elements such as iron (Fe) and manganese (Mn). This work has thus far developed a CO2 electrolysis system using Fe and Mn-based electrodes together with a silicon (Si) solar cell that promotes the CO2 reduction reaction with a solar conversion efficiency of over 10%.
- (1)Morikawa, T., et al., Applied Physics Letters, Vol. 98 (2011), 242108.
https://doi.org/10.1063/1.3599852 - (2)Sato, S., Saita K., Sekizawa K., Maeda S., Morikawa, T., ACS Catalysis, Vol. 8 (2018), pp. 4452-4458.
https://doi.org/10.1021/acscatal.8b01068 - (3)Suzuki, T. M., Nonaka, T., et al., Sustainable Energy & Fuels, Vol. 1 (2017), pp. 636-643.
https://doi.org/10.1039/c7se00043j - (4)Sekizawa, et al, ACS Catalysis, Vol.8 (2018), pp 1405-1416.
https://doi.org/10.1021/acscatal.7b03244 - (5)Arai, T., Sato, S., Sekizawa, K., Suzuki, T. M. and Morikawa, T., Chemical Communications, Vol. 55 (2019), pp. 237-240.
https://doi.org/10.1039/c8cc07900e
- (1)Morikawa, T., et al., Applied Physics Letters, Vol. 98 (2011), 242108.
- 5. Analyses of electronic states and electron transfer mechanism for semiconductor metal complex
-
CO2 reduction is a chemical reaction that consists of multiple steps, including light absorption, electron excitation, electron transfer and protonation. These processes determine the overall efficiency of the reaction and the selectivity for the various possible products. Unfortunately, the details of these reactions have not yet been fully clarified. We have therefore examined the associated mechanisms and the electronic structures of the photocatalysts using ultra-fast transient absorption spectroscopy, electronic structure analysis at a synchrotron radiation facility, and theoretical computations, while collaborating with experts both inside and outside the company. Combined theoretical and experimental investigations of a N-doped Ta2O5/Ru-complex hybrid capable of functioning as a CO2 reduction photocatalyst found that N doping raised the conduction band minimum of Ta2O5 and promoted the transfer of excited electrons to the Ru-complex mounted on the surface of the material.
- (1)Shirai, S.; Sato, et al., T., Journal of Physical Chemistry C, Vol. 122 (2018) pp. 1921-1929.
https://doi.org/10.1021/acs.jpcc.7b09670 - (2)Yamanaka, K., Sato, et al., T., Journal of Physical Chemistry C, Vol. 115 (2011), pp. 18348-18353.
https://doi.org/10.1021/jp205223k - (3)Sato, S., Onda, K., et al., Chemistry – A European Journal, Vol. 27 (2020), pp. 1127-1137.
https://doi.org/10.1002/chem.202004068 - (4)Morikawa, T., Gul, S., Nishimura, Y.F., Suzuki, T.M. and Yano, J., Chemical Communications, Vol. 56 (2020), pp. 5158-5161.
https://doi.org/10.1039/d0cc00692k
- (1)Shirai, S.; Sato, et al., T., Journal of Physical Chemistry C, Vol. 122 (2018) pp. 1921-1929.
- 6. Large reaction cells
-
A cooperating research team has begun efforts to accelerate the future implementation of artificial photosynthesis technologies in society. CO2 reduction with a high efficiency of 7.2% has been demonstrated in a system with an electrode area approximately 1,000 times larger than the that of monolithic element. <*Link to Kato's page>
News Release Solar-Driven CO2 Recycling Technology! Dramatic Breakthrough in Artificial Photosynthesis - 7. Contributing to society through elemental recycling technologies
-
At present, we are also conducting research on technologies intended to realize high throughput CO2 reduction for the synthesis of formic acid and carbon monoxide, and to demonstrate generation of molecules with chemical energy greater than them.